History
Scientists think that the ancestry of corn in the Americas dates back to 10,000 years ago, and that it is closely related to a wild grass called teosinte. Corn, squash and beans were once known as the "Three Sisters" by our native peoples - sisters who should never be apart - sisters who should be planted together. These three plants were important sources of food. In fact, corn was very important to the survival of the first English colonists during their first winters in Northeastern America. The survival of the early colonists depended on what corn they could beg, borrow or steal from the native peoples plus what they were able to grow under their guidance.
It is believed that corn dates back even further than the inhabitance of native people. Corn's origin is believed to be in the Mexican plateau or the highlands of Guatemala. Fossil pollen grains of corn have been found in drill cores of lake sediment beneath Mexico City. These sediments could be 80,000 years old or more.
Corn belongs to the grass family. Theory suggests that at one time, each individual kernel was covered by its own floral parts similar to the kernels of oats and barley, and that the cob readily broke down into small segments. It is believed that this has allowed corn as a species to survive. The husk and cob as we know them today were gradually developed from wild varieties by the native population.
When Jacques Cartier visited the village of Hochelaga (now Montreal) in 1535, he noted the extensive corn fields growing in all directions. There are records of Champlain finding corn growing in the area of Georgian Bay in 1615. Archeological studies have found that corn was grown near Campbellville, Ontario before 1200 A.D.
Native groups developed the major classes of corn that we recognize today, the types being sweet, popping, flint, flour and dent corns. Dent corn usually produces higher grain yields and to this day dominates production in North America and much of the rest of the world.
From its original use as a food for man, corn now yields over one hundred by-products to industry. A few of the by-products are dyes, paints, oilcloth, oil for soaps, syrups, starches, size and glaze, corn gum (used as a rubber substitute), vegetable substitutes for lard and butter, corn cellulose in press boards and insulating materials and various chemicals. The livestock industry continues to be the largest user of corn grown. Approximately 60% of the crop harvested is fed to livestock. Approximately 30% is used for industrial and commercial uses which leaves about 10% for the export market. Research continues to find new uses for corn. Environmentally friendly products are one such area. Corn as a renewable resource can be beneficial in making products such as ethanol fuel, ethanol windshield washer fluid, CMA (calcium magnesium acetate) road de-icer, and other degradable products made from corn starch.
Current Farming Practices: Tillage
Recent research has shown that soil tillage ("working" of the soil by farm and garden implements) has a major effect on the rate of soil organic matter decomposition. More tillage means faster rates of organic matter oxidation (breakdown). Conversely, the techniques of minimum tillage and "no-till" (also called zero tillage and no tillage) mean slower rates of organic matter decay.
In a University of Guelph experiment comparing different tillage practices for corn, the topsoil (0-15 cm depth) of plots devoted to no-till corn production had an average of 19.8 tonnes/hectare more organic matter after 18 years of research than did plots where corn was grown using traditional tillage (fall moldboard plowing plus secondary tillage in springtime) methods (T.J. Vyn, Department of Crop Science, referenced in Ontario Corn Producer, February 1994). (See table below. There was no difference among tillage treatments in this experiment in soil organic matter levels at lower depths in the soil.)
Tillage System |
Soil Organic Matter (tonnes/hectare) |
Zero tillage |
86.2 |
Fall chisel plow |
73.1 |
Offset disc |
74.3 |
Spring mold board plow |
74.8 |
Fall mold board plow |
66.4 |
Least significant difference (P=0.05) |
13.8 |
Figure 1: Soil organic matter levels after 18 years of various tillage systems on a silt loam soil near Elora, Ontario. |
Other research results have shown that soil organic matter levels can be expected to increase as corn yield levels increase.
Traditional corn production practices, characterized by high amounts of soil tillage and relatively low crop yields, often meant decreases in soil organic matter percentages. The combination of newer, higher-yielding hybrids, and the use of minimum or no-till techniques, should mean the reverse. The greatest reductions in soil organic matter levels occur when soil is tilled regularly during the summer with no crop being produced - the traditional summer fallowing practice . Surprisingly, many people still believe, erroneously, that such a "resting process" is good for the soil, and, also, that cropping programs which produce high yields of organic matter are inherently "bad."
Pesticide Use
The quantity of pesticides used per hectare of land planted to corn is similar to that of major spring-seeded crops. Per tonne of crop yield, the rate of usage tends to be lower with corn. The quantity of pesticide usage per hectare and the real expenditure for pesticide purchases is trending downward for corn and other major field crops in Ontario. This trend to reduced rates is occurring despite a reduction in the amount of soil tillage. (Soil tillage is a "traditional," non-chemical method of weed control.) Public concerns with respect to pesticide usage in agriculture are two-fold: the risk of food contamination, and the risk of contamination of water, soil and air.
Corn Refining Process
Corn refining is today's leading example of value added agriculture. The nine member companies of the Corn Refiners Association, Inc. use over 1.4 billion bushels of U.S.-grown corn to produce a broad array of food, industrial and feed products for Americans and for the world market. Corn refiners use shelled corn which has been stripped from the cob during harvesting. Refiners separate the corn into its components -- starch, oil, protein and fiber -- and convert them into higher value products.
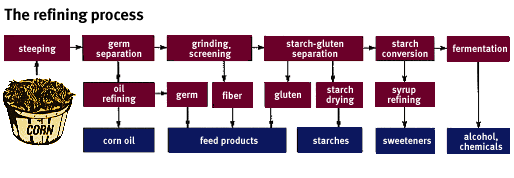
Figure 2: A diagram of the refining process1
Corn sweeteners are the most important refined corn products. Last year, corn sweeteners supplied more than 55 percent of the U.S. nutritive sweetener market. The second major refined corn product is ethanol, which is gaining increasing acceptance as a cleaner burning option for motor fuels. The third major corn product -- a mainstay of the industry and of the U.S. economy -- is starch. Americans rely on corn refiners for over 90 percent of their starch needs. Corn refining is America's premier bioproducts industry, with increasing production of amino acids, antibiotics and degradable plastics adding further value to the U.S. corn crop. In addition to starches, sweeteners and ethanol -- all made from the starch portion of the corn -- refiners produce corn oil and a variety of important feed products.
Corn refining began in the United States around the time of the civil war with the development of the process for cornstarch hydrolysis. Prior to this time, the main sources for starch had been wheat and potatoes. In 1844, the Wm. Colgate & Company wheat starch plant in Jersey City, NJ, became the first dedicated cornstarch plant in the world. By 1857, the corn starch industry reached significant proportions in the U.S. Starch was the only product of the corn refining industry. Its largest customer was the laundry business.
The next major event in the history of corn refining was the production of dextrose from cornstarch in 1866. This industrial application and subsequent developments in the chemistry of sugars served as early scientific links to a growing body of starch technology. Other product developments in corn sweeteners took place more than 15 years later with the first manufacture of refined corn sugar or anhydrous sugar in 1882. Around the same time, the industry also began to realize the value of the non-starch parts of corn. Fiber, germ and protein from the corn had simply been discarded until manufacturers discovered they could turn them into valuable animal feed ingredients. Corn gluten feed was first manufactured in 1882. The industry then discovered that corn oil could be extracted from the germ. The first commercial production of corn oil took place in 1889.
Then in 1893, the industry began to recover the steepwater used to release the starch in the refining process and condense it to use in feed products. Major strides in starch chemistry and the introduction of modified food starches, as well as many important process refinements took place in the early 1900's. In 1900, the manufacture of thin boiling starches was introduced and chlorinated starches were introduced about 15 years later.
Corn syrup technology advanced significantly with the introduction of enzyme-hydrolyzed products. In 1921, crystalline dextrose hydrate was introduced. Then in the mid-1950's, the technology for commercially preparing low conversion products such as maltodextrin and low DE syrups was developed. The purification and crystallization of dextrose meant for the first time that corn-based sweeteners could compete in some markets that had been the sole domain of the sugar industry.
The next developments involved enzyme-catalyzed isomerization of dextrose to fructose. The first commercial shipment of high fructose corn syrup (HFCS) took place in 1967. The fructose content of the syrup was around 15 percent. Further research enabled the industry to develop a higher conversion and the first commercial shipment of HFCS-42 or 42 percent fructose syrup took place a year later. Further refinements in the process were developed in the late 1970s and by the mid-1980s, HFCS became the sweetener of choice for the soft drink industry in the U.S.
The production of ethanol by corn refiners began after World War II, but major quantities were not manufactured until the 1970's. Several corn refiners began fermenting dextrose to make beverage and industrial alcohol. Corn refiners' entry into the fermentation business is key to some of the major changes the industry will see in the future. The industry began to develop an expertise in industrial microbiology.
Today, starch, glucose and dextrose are still core products of the wet milling industry. But the products of microbiology fructose, ethanol, food additives and chemicals have overshadowed them. New technology and research has significantly expanded the industry's product portfolio. Advances in process engineering and biotechnology have enabled refiners to become low-cost suppliers of basic food and chemical ingredients opening new markets in the food and industrial sectors. Over the past couple of decades major developments of the industry include ethanol as a motor fuel, HFCS reaching a near-par with sugar consumption, specialty sweeteners such as crystalline fructose, food and feed additives such as vitamins, lysine, tryptophan and others, organic acids for industrial and food use, and degradable replacements for chemical products. Corn refiners will continue this dynamic product evolution by combining technologies rooted in the history of the industry with new technologies from outside the industry to create new markets and greater product diversity.
Wet Milling
The wet milling process yields approximately 31.5 pounds of starch, which can be further processed into 33 pounds of sweetener or 2.5 gallons of ethanol. In addition, 13.5 pounds of corn gluten feed, 2.5 pounds of corn gluten meal and 1.6 pounds of corn oil can be extracted. The ethanol made from one bushel of shelled corn can provide 197,000 to 220,400 BTUs depending on the recovery rate.
For more than 150 years, corn refiners have been perfecting the process of separating corn into its component parts to create a myriad of value added products. The corn wet milling process separates corn into its four basic components: starch, germ, fiber and protein.
There are five basic steps to accomplish this process. First the incoming corn is inspected and cleaned. Then it is steeped for 30 to 40 hours to begin breaking the starch and protein bonds. The next step in the process involves a coarse grind to separate the germ from the rest of the kernel. The remaining slurry consisting of fiber, starch and protein is finely ground and screened to separate the fiber from the starch and protein. The starch is separated from the remaining slurry in hydrocyclones. The starch then can be converted to syrup or it can be made into several other products through a fermentation process.
Refinery staff inspect arriving corn shipments and clean them twice to remove cob, dust, chaff and foreign materials before steeping, the first processing step, begins. Corn refining has been the fastest growing market for U.S. agriculture over the past twenty years, and refiners now use around 15% of the $25 billion U.S. corn crop. Since a large amount of the nations' corn production never leaves the farm on which it was produced, corn refining is a vital factor in the cash market for U.S. corn. Each day the production of about 33 thousand acres of corn arrives at corn refining facilities before conversion to food, industrial and feed products.
Each stainless steel steep tank holds about 3,000 bushels of corn for 30 to 40 hours of soaking in 50-degree water. During steeping, the kernels absorb water, increasing their moisture levels from 15 percent to 45 percent and more than doubling in size. The addition of 0.1 percent sulfur dioxide to the water prevents excessive bacterial growth in the warm environment. As the corn swells and softens, the mild acidity of the steepwater begins to loosen the gluten bonds within the corn and release the starch. After steeping, the corn is coarsely ground to break the germ loose from other components. Steepwater is condensed to capture nutrients in the water for use in animal feeds and for a nutrient for later fermentation processes. The ground corn, in a water slurry, flows to the germ separators.
Cyclone separators spin the low-density corn germ out of the slurry. The germs, containing about 85% of corn's oil, are pumped onto screens and washed repeatedly to remove any starch left in the mixture. A combination of mechanical and solvent processes extracts the oil from the germ. The oil is then refined and filtered into finished corn oil. The germ residue is saved as another useful component of animal feeds.
The corn and water slurry leaves the germ separator for a second, more thorough, grinding in an impact or attrition-impact mill to release the starch and gluten from the fiber in the kernel. The suspension of starch, gluten and fiber flows over fixed concave screens, which catch fiber but allow starch and gluten to pass through. The fiber is collected, slurried, and screened again to reclaim any residual starch or protein, then piped to the feed house as a major ingredient of animal feeds. The starch-gluten suspension, called mill starch, is piped to the starch separators.
Gluten has a low density compared to starch. By passing mill starch through a centrifuge, the gluten is readily spun out for use in animal feeds. The starch, with just one or two percent protein remaining, is diluted, washed 8 to 14 times, rediluted and washed again in hydrocyclones to remove the last trace of protein and produce high quality starch, typically more than 99.5 percent pure. Some of the starch is dried and marketed as unmodified cornstarch, some is modified into specialty starches, but most is converted into corn syrups and dextrose.
Starch, suspended in water, is liquefied in the presence of acid and/or enzymes that convert the starch to a low-dextrose solution. Treatment with another enzyme continues the conversion process. Throughout the process, refiners can halt acid or enzyme actions at key points to produce the right mixture of sugars like dextrose and maltose for syrups to meet different needs. In some syrups, the conversion of starch to sugars is halted at an early stage to produce low-to-medium sweetness syrups. In others, the conversion is allowed to proceed until the syrup is nearly all dextrose. The syrup is refined in filters, centrifuges and ion-exchange columns, and excess water is evaporated. Syrups are sold directly, crystallized into pure dextrose, or processed further to create high fructose corn syrup.
Dextrose is one of the most fermentable of all of the sugars. Following conversion of starch to dextrose, many corn refiners pipe dextrose to fermentation facilities where the dextrose is converted to alcohol by traditional yeast fermentation or to amino acids and other bioproducts through either yeast or bacterial fermentation. After fermentation, the resulting broth is distilled to recover alcohol or concentrated through membrane separation to produce other bioproducts. Carbon dioxide from fermentation is recaptured for sale and nutrients remaining after fermentation are used as components of animal feed ingredients.
Hybrid Corn
A major change occurred in the 1930s and early 1940s when the use of hybrid corn became prevalent. The new hybrids were much higher yielding, more competitive with weed species, and much more tolerant of the European corn borer - an insect pest which wrecked havoc on corn fields in earlier years.
The introduction of hybrids meant that farmers were no longer able to use seed harvested from their own fields to plant the next year's crop (unless, of course, they were willing to accept the low yields and other problems associated with the use of "open-pollinated" corn varieties), and were obliged to buy new hybrid seed from seed companies each year.
This annual purchase of hybrid corn seed, in turn, financed a major investment in private corn breeding. As a result, yields of corn have generally increased at a faster rate over the past 30-40 years, than those of other, non-hybrid crops. Major improvements have also been made in other traits such as standability (the ability to remain standing until time of harvest), early maturity (permitting hybrid corn to be grown in much cooler, shorter-season areas of Canada than was formerly the case), disease resistance, insect tolerance, grain quality, and the rate of pre-harvest grain drying (thereby reducing the cost of artificial drying after harvest).
Newer hybrids are more tolerant of drought and shading. Indeed, newer hybrids are more tolerant of many stresses than were their predecessors, including the indigenous varieties grown centuries ago. These improvements include greater resistance or tolerance to weed competition, low fertility, shading and lodging.
Public research has been important in developing inbreds and broadly based breeding "populations" which can be used by commercial breeders for hybrid development. Public researchers have also helped private breeders in their search for greater pest resistance and/or tolerance, and in the improvement of grain quality, lodging resistance, and crop yield.
Major effort is being made, internationally, to preserve indigenous populations of corn for potential use in future breeding programs. Those who maintain these populations (this means growing plants and increasing seed supply from time to time, under conditions which ensure that these plants are not cross-fertilized with pollen from corn plants in adjacent fields) include universities, Agriculture Canada, the United States Department of Agriculture, the Centro Internacionale de Mais y Trigo in Mexico, and most major private seed companies. An estimated 50,000 different types of corn exist in "genebanks" around the world. There are about 250 to 300 races of corn in existence, of which only a few are used extensively for commercial production.
Biotechnology offers the potential for further improvement in corn. Features which might be improved or added to corn, with potential for improvements in both farm income and environmental quality, include complete genetic resistance to insects such as the European corn borer and corn rootworm, nitrogen fixing ability, greater resistance to stalk and root rot disease organisms, tolerance to high and low temperatures, and faster rates of natural grain drying. Higher yield potential, with its associated benefit of increasing soil organic matter levels, remains an important breeding objective.
Environmental Impact
Corn produces high yields of organic matter (biomass) per hectare of land area per year, relative to most other plant species, because it has a highly efficient photosynthetic system and a long period of seasonal growth. The annual biomass production of a typical corn crop is about double that of an average temperate-climate forest. An average hectare of corn produces enough oxygen per hectare per day in mid summer to meet the respiratory needs of about 325 people.
A portion of the organic matter produced by corn is removed as the harvested crop. With grain corn production, the harvested portion represents about 40% of all dry matter produced with 60% being left on or in the soil after harvest. With forage corn, where the entire above-ground plant is harvested, the ratio is about 80% removed: 20% left. Where the harvested corn is used to feed livestock animals, about 1/5 to 1/3 of the harvested biomass is returned to the soil in the form of manure. With manure composting, the amount of organic matter returned to the soil is less. (About half of the organic matter in manure is converted back into carbon dioxide during composting.) Some of the byproducts of industrial corn processing, particularly those components high in protein such as "corn gluten feed" and "distillers grains," are also used as feed, and are returned, in part, to the soil as livestock manure.
The organic matter returned to the soil, directly from crop residues or indirectly as manure, consists of many different organic compounds. Some of these are digested quickly by soil microorganisms. The result of this is a rapid formation of microbial compounds and body structures - important in holding soil particles together to provide soil structure and limit soil erosion - and the release of carbon dioxide back to the atmosphere through microbial respiration.
Many of the organic compounds returned to the soil after corn harvest, are digested more slowly by soil organisms. Some of these compounds don't break down for many years. Longer- lasting compounds, such as lignin, also help preserve and improve soil structure by creating pores for air penetration, by holding soil water, and by helping soil resist the compaction caused by field equipment, tramping animals, and rainfall. The rate of decomposition of dead corn plants is slower than for many other plants - this is one reason why corn stalks left on the surface provide good long-term protection against wind and rainfall.
Soil nutrients, particularly potassium, phosphates, and nitrogen - the latter in the form of either nitrate or ammonium - are needed for corn growth and development. The fertility needs of corn tend to be higher, when expressed on per-hectare basis, than those of other crops. When fertilizer needs are expressed on a per-tonne-of-production basis, however, fertility needs for corn are similar to or lower than those of other grain crop species.
Plant nutrient needs can be supplied by a reduction in soil nutrient levels and organic matter breakdown - processes sometimes called "soil mining" - or by the application of organic or inorganic fertilizers. Organic fertilizer materials (for example, manure, compost, "sludge" from municipal waste treatment, and crop residues) must be broken down by soil microorganisms to produce inorganic fertilizer ions - the same ones supplied by commercial "inorganic" fertilizers - before they can be taken up by plant roots.
If nutrients are available in excess of plant needs, the result can be the contamination of subsurface water, especially with mobile nutrients such as nitrate. However, if insufficient fertility is available, the result is lower rates of organic matter production by the crop and lower yields.
Soil testing can be used to predict, with good accuracy, fertilizer application needs in the case of potassium and phosphate. Techniques have been recently introduced which should permit similar accuracy with soil nitrate-nitrogen tests, although further research on soil nitrate-nitrogen testing is still required.
Nitrates in ground water may represent one of the largest environmental problems associated with agriculture. High soil nitrate levels can result from the use of manure or synthetic fertilizers, or from the vigorous growth of high-nitrogen-fixing legumes such as alfalfa. The challenge is to develop and perfect techniques which will enable farmers to meet the fertility needs of crop plants while reducing the risk of ground water contamination.
The increased use of crop rotations in which corn follows perennial legumes crops, such as alfalfa and red clover, provides a means of using the high nitrogen-fixing ability of the latter to supply part or all of the nitrogen fertility needs of the corn crop. While all corn varieties produce lower yields when insufficient fertility is available, higher yields are obtained with newer, rather than older, corn hybrids under conditions of low soil fertility, because newer hybrids use nitrogen more efficiently. Newer hybrids are more tolerant of many stresses than were their predecessors, including the indigenous varieties grown centuries ago. These improvements include greater resistance or tolerance to weed competition, low fertility, shading and lodging.
Fossil-fuel energy is used to produce corn. However, analyses show that the energy output:input ratio with corn - i.e. amount of energy contained in harvested grain relative to the amount of fossil-fuel energy used for its production, including the production of inputs and equipment - is approximately 5.5:1. The energy ratio for corn compares favourably with that of other crops.
As a user of atmospheric carbon dioxide, fossil fuels, and nitrogen fertilizers, corn does affect global net emissions of greenhouse gases. These gases are considered by many to represent a potential threat to global climate. However, during a full growing season, an average hectare of corn removes 22 tonnes of carbon dioxide from the air. Growing one million hectares of corn removes an annual quantity of carbon dioxide equivalent to that produced in burning about 9 billion litres of gasoline. Carbon dioxide is released to the atmosphere in manufacturing inputs (fertilizer, fuel, pest control products, equipment) used in growing corn. It can be estimated that about 1.3 tonnes of carbon dioxide are released during the production of a hectare of corn. This includes input manufacturing and all transportation costs. The ratio of carbon dioxide absorbed to carbon dioxide released is therefore about 17:1.
The ultimate carbon balance with corn production depends upon the fate of the organic matter produced by corn during a growing season. With grain corn, some of the organic matter produced may be reconverted back into carbon dioxide relatively quickly, for example in the production and digestion of food, or the manufacture and combustion of fuel ethanol. With other uses, for example the production of paper, glue and wallboard, the reconversion may not occur for many years, decades, or centuries.
As outlined earlier, at least 60% of the dry matter produced by a grain corn crop is returned to the soil after harvest. With conventional tillage methods, the rate of oxidation of soil organic matter was at least as great as the rate of organic matter addition, with the result being no net, long-term absorption of atmospheric carbon dioxide. Past research has shown that the balance depended on yield level, with corn providing a net addition to soil organic matter (with conventional tillage) when the amount of corn stalk dry matter returned to the soil exceeded 6 tonnes/ha. Depending upon assumptions made as to the relative contribution of corn root, stalk, leaf and cob residues to soil organic matter, this figure equates to about 6 tonnes/ha of grain corn yield (about 100 bu/acre). The trend to minimum tillage and no tillage can be expected to have even greater effects on the net carbon dioxide balance with corn production. The 19.8 tonnes/hectare increase in soil organic matter content of the topsoil of "no-till" versus conventionally tilled corn plots measured after 18 years of experimentation at the University of Guelph equates to about 42 tonnes of carbon dioxide per hectare of soil surface. This is equivalent to that quantity of carbon dioxide released in combusting over 18,000 litres of gasoline.
The U.S. Environmental Protection Agency (1991) has calculated that a shift from 27% conventional tillage in U.S. agriculture in 1990 to 76% conservation tillage by 2010 would mean a net increase of about 272-444 million tonnes in the soil organic carbon content (equivalent to 1.0- 1.6 billion tonnes of carbon dioxide) of U.S. farm fields by the year 2020. The same report concludes, "The release of soil carbon globally from agriculture has been estimated at 800 Tg [1 Tg = 1 million tonnes] C per year. If alternative tillage practices could prevent this loss, then approximately 16% of the annual global fossil fuel emissions [5,300 Tg C per year] would be offset without considering sequestration of carbon in soils with management practices such as no- till agriculture. Other benefits would accrue since [soil organic carbon] has a positive influence on soil fertility, structure, water infiltration and heat flow, water holding capacity, aeration, size and distribution of water-stable aggregates, and reduce soil erosion." Wallace et al. (1990) stated that "a no-till cropping system may be a most important procedure for solving the world greenhouse problem."
As corn yield levels continue to increase and as tillage and input usage decline, the importance of corn as a "sink" for carbon dioxide should increase substantially.
Nitrous oxide (N2O), a greenhouse gas, is released to the atmosphere during the production of nitrogen fertilizer. Nitrous oxide may also be released by soil micro-organisms during the natural reduction or oxidation of organic and inorganic nitrogen fertilizer materials. Environment Canada (Jaques, 1992) has estimated that about 12 thousand tonnes of N2O are released annually in Canada as a result of fertilizer manufacturing and usage. If it is assumed (based on Agriculture and Agri-Food Canada data) that about 10% of Canadian nitrogen fertilizer usage is for corn, this equates to about 1 kg of N2O per hectare of land seeded to corn. One kg of atmospheric N2O has the same "global warming potential" as about 290 kg of carbon dioxide (Intergovernmental Panel on Climate Change, 1990). Although this is a relatively small amount in proportion to the amount of carbon dioxide removed from the air during normal production, opportunity exists to reduce N2O losses through increased efficiency of usage of nitrogen fertilizer.
Ethanol made from corn and other renewable, biological feedstocks is used extensively as an automotive fuel. Ethanol represents a means of reducing net additions of atmospheric carbon dioxide emissions associated with transportation fuel usage. Ethanol represents an environmentally attractive substitute for lead, methylcyclopentadienyl manganese tricarbonyl (MMT), benzene and other undesirable compounds used as octane enhancers in gasoline. Ethanol represents a renewable, home-grown means of reducing dependence on imported crude oil.
Sustainable development was defined by the World Commission on Environment and Development (the "Bruntland Commission") as "development which meets the needs of the present without compromising the ability of future generations to meet their own needs." Corn production includes features which could be classed as sustainable, and others which are not. Sustainable features include the use of sunshine, carbon dioxide and rainfall as the principal ingredients for corn growth. With conservation tillage techniques and higher corn yields, future soil organic matter levels can be expected to increase when land is planted to corn. Efficient use of livestock manure represents one means of reducing the need for synthetic nitrogen fertilizers. A second involves the use of high-nitrogen-fixing crops such as alfalfa in the crop rotation, although this can only occur if there is a use for the legume forage produced. Biotechnology may provide a solution if researchers are able to transfer nitrogen-fixing abilities, genetically, from legume species into corn.
The decline in soil organic matter levels associated with older methods of corn production could be classed as non sustainable. However, with conservation tillage techniques and higher corn yields, future soil organic matter levels can be expected to increase when land is planted to corn. Conservation tillage and crop rotations which leave the soil surface protected against the action of wind, rainfall, and melting snow have markedly reduced the amount of soil erosion traditionally associated with corn production.
Pesticides (mostly herbicides in the case of corn production) are currently made from petroleum, a non-renewable resource. However, the quantities used are low - generally 2 to 3 kg/hectare of active ingredient - and declining. The same situation exists for fossil fuels. An average of about 53 litres of petroleum-based fuels per hectare are used in field operations to grow corn. This quantity is declining. The U.S. Environmental Protection Agency has estimated that fossil fuel usage in field crop production can be reduced by almost 50% with no-till technology.
The technology exists to use ethanol made from corn, or "soy-diesel" made from soybeans, as on-farm fuels. Refuse from corn production - i.e. corn cobs and husks - can be, and is being, used as a fuel for grain drying and for on-farm heating. The extent to which these uses of renewable fuel energy in agriculture expand will depend on the efficiencies of new technology and economics.
There are no effective substitutes for potassium and phosphate fertilizers used in the production of corn - and virtually all other agricultural crops. The supply of available potassium is enormous - sufficient to meet predicted market needs for at least many centuries - and the energy cost needed for mining, transportation and application is small. With phosphate fertilizer, large global reserves of rock phosphate exist, especially in the southeastern United States - but some energy is needed to process this rock into available plant fertilizer. (The availability of phosphate to plants from rock phosphate is very low.) Corn biomass energy could be used to reduce rock phosphate, but this is not likely to occur unless the price of non-biomass energy becomes significantly higher. Fortunately, the annual phosphate fertility requirement by corn, and energy used in meeting this requirement, is generally smaller than for potassium or nitrogen.
The manufacture of nitrogen fertilizer, using natural gas as the energy source and atmospheric nitrogen gas as the feedstock, represents about half of the fossil-fuel energy requirement for corn production. A similar situation exists for most non-legume, high-yielding farm crops. Efficient use of livestock manure represents one means of reducing the need for synthetic nitrogen fertilizers. A second involves the use of high-nitrogen-fixing crops such as alfalfa in the crop rotation, although this can only occur if there is a use for the legume forage produced. This generally means production of ruminant livestock animals such as cattle and sheep. Annual legumes such as soybeans, field beans, and even forage legumes grown as a part- season cover crop (for example, red clover after winter wheat) normally can provide only a modest percentage of the soil nitrogen compounds needed for successful production of corn the following year.
Non-agricultural products, for example urban wastes, could potentially serve as a source of nitrogen fertilizer. However, health hazards (to both plants and humans) can exist with this material, and procedures commonly used to reduce these hazards - such as composting - tend to reduce the fertilizer value. (About half, or more, of the nitrogen in plant wastes may be lost by leaching or volatilization during composting.)
Biotechnology may provide a solution if researchers are able to transfer nitrogen-fixing abilities, genetically, from legume species into corn. Until this occurs, the requirement for nitrogen fertilizers will remain the least sustainable feature of corn production, given the impracticality and environmental risks of supplying all of the crop's needs using animal manure, perennial legumes or urban wastes as dominant sources of nitrogen supply. The combustion of biomass as an energy source for nitrogen fixation also seems impractical, given the size of the need, and the high scale efficiencies associated with nitrogen fertilizer manufacture in large, "world-scale" plants.
It should be noted that the limiting resource is energy, not supply of nitrogen gas. Global atmosphere is almost 80% nitrogen gas! The source of fossil energy for nitrogen fertilizer manufacture is natural gas, and natural gas reserves are relatively large - unlike those for light crude petroleum. This dependence on synthetic nitrogen fertilizers is not unique to corn. The requirement per tonne of grain or seed produced is similar for most major non-legume crops. Technology now being developed to permit the times and rates of nitrogen application to be more closely tailored to individual crop needs, should permit the overall need to be reduced significantly - though not eliminated.
References
- Corn Refiners Association (corn.org)